2021 Excellence in Environmental Engineering and Science® Awards Competition Winner
Honor Award - University Research
Locally Enhanced Electric Field Treatment (LEEFT) for Disinfection
Entrant: Xing, Xie, Ph.D. Person in Charge: Xing, Xie, Ph.D. Location: Atlanta, Georgia Media Contact: Xing, Xie, Ph.D.
Entrant Profile
Dr. Xing Xie is an assistant professor and the Carlton S. Wilder Junior Professor in the School of Civil and Environmental Engineering at Georgia Tech. Prior to joining Georgia Tech, he was a postdoc at Caltech in 2014-2017. Dr. Xie received his B.S. (2006) and M.S. (2008) degrees in Environmental Science & Engineering from Tsinghua. He received his Ph.D. (2014) in Civil & Environmental Engineering and his second M.S. degree (2012) in Materials Science & Engineering from Stanford.
Dr. Xie’s research interests center around the applications of environmental biotechnology and materials science at the nexus of water and energy. He has worked on many projects related to water treatment and reuse, pollutant detection and quantification, and energy and resource recovery. He has published 65 peer-reviewed articles in leading journals, including PNAS, Nature Communications, and Environmental Science & Technology. His work has been cited over 6500 times with an H-index of 25.
Dr. Xie is a recipient of the National Science Foundation CAREER Award, the Sustainable Nanotechnology Organization Emerging Investigator Award, Georgia Tech Paul A. Duke GIFT Action Plan Achievement Mentor Award, and Georgia Tech Bill Schutz Junior Faculty Teaching Award.
Dr. Xie is the principle investigator of the project entered, and his roles include directing research, supervising students, analyzing results, and preparing reports. Students in Dr. Xie’s group involved in this project include Jianfeng Zhou, Ting Wang, Cecilia Yu, Mourin Jarin, Peirui Liu, Beichen Lin, Zhengyang Huo, Nissim Gore-Datar, Naishee Kamdar, Manhitha Jumili, and Betty Sui.
Project Description
Fig. 1 Schematic of LEEFT. (A) Electric-field enhancement by a co-axial electrode design; (B) Electric-field enhancement by microscale structures with sharp tips on the electrode surface.
Fig. 2 The rational design of coaxial-electrode LEEFT device. (A) 3D schematic shows the device set-up. (B) Scanning electron microscopy images show the center copper-oxide-nanowire-modified copper electrode. (C) Electric-field simulation on the cross-section of the device showing the non-uniform distribution of the electric field with a two-level strength enhancement."
Fig. 3 Coaxial-electrode LEEFT disinfection performance. (A) Comparison of the disinfection performances of E. coli under different applied voltage showing LEEFT can operate at a low voltage. (B) Long-term treatment against E. coli showing a stable and superior disinfection performance for 16h. (C) Inactivation efficiencies of E. coli in the river water, Enterobacter, Bacillus subtilis, and Staphylococcus epidemidis with varied flow rates and fixed voltage (1V). (D) E. coli inactivation performance in a 6-feetlong scaled-up LEEFT device. Insertion shows the photographs of the scaled-up device.
Complexity.
Over 10% of the world’s population lack access to safe drinking water. Waterborne diseases caused by pathogenic microorganisms result in ~2,000 death every day, significantly reducing the life expectancy in developing countries. Apparently, effective water disinfection methods are not readily accessible to these people.
In developed areas, water is typically disinfected in centralized facilities. The most commonly applied chlorination effectively inactivates most pathogens in water, but inevitably generates harmful disinfection byproducts (DBPs). Such a dilemma has been bothering environmental engineers since the 1970s. Alternative disinfection methods have been developed, but applications are limited by microbial regrowth, lack of lasting disinfection capability, and/or high operation cost.
Originality and Innovation.
We have developed a novel disinfection technology called locally-enhanced-electric-field treatment (LEEFT), which uses a strong electric field to assist or directly induce microbial inactivation. When microorganisms are exposed to an external electric field, the transmembrane potential across cell membranes will be elevated. This phenomenon increases membrane permeability or, with a sufficiently strong electric field (e.g., >10kV/cm), generates pores on the membrane that can cause direct inactivation.
Microbial inactivation by EFT is a physical process without consuming chemicals and generating DBPs. When a sufficiently strong electric field is provided, EFT can be extremely fast (e.g., 6-log inactivation within 100 microseconds). EFT is also distinct from electrochemical processes where inactivation is due to generated microbicidal chemicals and/or direct oxidation. To achieve a sufficiently strong electric field, conventional EFT (CEFT) applies a high voltage (e.g., >10kV) across two parallel plate electrodes with a short distance (e.g., <1cm). CEFT has been mainly used for biomedical applications because of high energy consumption and low throughput. Dramatically different from CEFT, LEEFT overcomes these hurdles and thus becomes economically feasible for water disinfection.
The design goal of CEFT is to achieve a uniform electric field and avoid dead zones so that all microorganisms in the system can be inactivated simultaneously. Differently, LEEFT aims to enhance the electric field locally so that microbial inactivation can be achieved in these regions with lower voltages applied (e.g., <10V), and to transport microorganisms to these regions by various forces (e.g., hydrodynamic, electrophoresis, and dielectrophoresis) to realize high-efficiency disinfection.
Quality.
To promote LEEFT, we have worked on the system development and performance optimization for water disinfection. In the past five years, we have published fifteen research articles and filed two patent applications on LEEFT.
We have realized local electric- field enhancement using two strategies. First, at the macroscale, co-axial electrodes (instead of parallel electrodes for CEFT) can be applied in tubular LEEFT devices (Fig.1A). With this configuration, the electric field is stronger when closer to the center electrode. Secondly, the electrode surface can be modified by microscale structures with sharp tips (Fig.1B). Attributed to the “lightning-rod” effect, the electric field near the tips is significantly stronger. Prototypes have been constructed, and the simulation results show that the electric-field strength can be enhanced by at least 3-4 orders of magnitude, which enables microbial inactivation with low voltages (Fig.2).
We have demonstrated that LEEFT alone can achieve high-efficiency disinfection (Fig.3). When both synthetic and natural water samples dosed with model bacteria, inactivation efficiencies of >6logs have been realized with applied voltages as low as 1V. Mild LEEFT (e.g., lower electric-field strength or faster treatment) has been applied synergetically with conventional disinfectants such as ozone and copper. The doses of conventional disinfectants to achieve the same level of disinfection can be significantly reduced (e.g., 6-log inactivation only requires 0.2mg/L copper, much lower than EPA’s secondary drinking water standard of 1.0mg/L).
An Integrated Approach.
Next-generation water disinfection should minimize the use of chemicals, the consumption of energy, and the impact on the environment, while having high resilience for different application scenarios. As a physical process, LEEFT neither releases harmful chemicals to the environment (water, soil, and atmosphere) and eco- system, nor generates solid waste (e.g., sludge and polymeric membranes) that requires disposal.
With a great potential to transform current water disinfection strategies and systems, LEEFT can potentially be applicable at all scales, from portable devices to point-of-use household units and from distributed community-scale clusters to centralized plants. LEEFT is especially attractive for small drinking water systems that may be close to residential areas, have difficulties in chemical delivery and storage, or lack knowledgeable operators. In traditional systems with centralized disinfection, LEEFT can be applied for “secondary disinfection” to maintain the water quality in the distribution system without residual chlorine. For remote places without grid power (e.g., islands, ships, submarines, space stations, and developing areas) or emergency situations when the grid power is disrupted (e.g., earthquakes and hurricanes), LEEFT can also be easily powered by batteries or renewable energy sources.
Besides water disinfection, LEEFT also has other potential applications attributed to the same antimicrobial mechanism. For example, we have demonstrated that LEEFT can be a promising approach to control algae bloom caused by eutrophication. In addition, we have been trying to use LEEFT for air disinfection, whose importance has been shown in the COVID-19 pandemic.
Economic and Social Impacts.
LEEFT disinfection is an energy-efficient process with a specific energy consumption of 1~100J/L, compared to 20-60J/L for UV, 50-100J/L for ozone, and 500-5000J/L for membrane processes. When combined with copper disinfection, the LEEFT-copper has an estimated overall cost (including both material and energy) of $0.10/m3, compared to $0.84/m3 for ozone and $0.68/m3 for chlorine. The global water disinfection market is projected to reach US$250 billion by 2024. Therefore, implementing LEEFT in the future will have a significant economic impact.
Because of the high efficiency, low cost, and simple operation, we expect LEEFT will be easily accepted by the public and adopted by the market in the future. Currently, over 1.1 million Americans lack a piped water connection, and more than 250 million Americans drink tap water that contains potentially carcinogenic DBPs. LEEFT will provide a more accessible and reliable alternative for water disinfection, which will enhance the protection of public health. Such social impact can be extended to the 800 million people worldwide that lack access to clean drinking water.
Click images to enlarge in separate window.
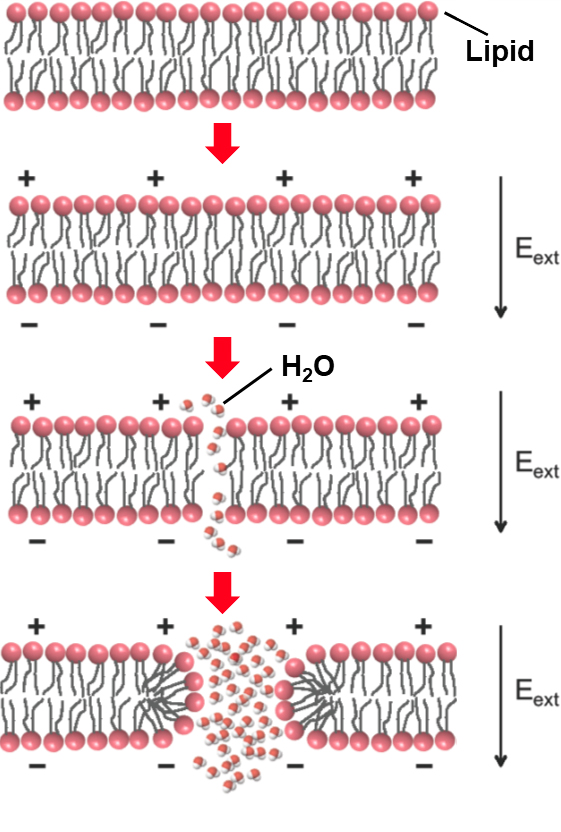 |
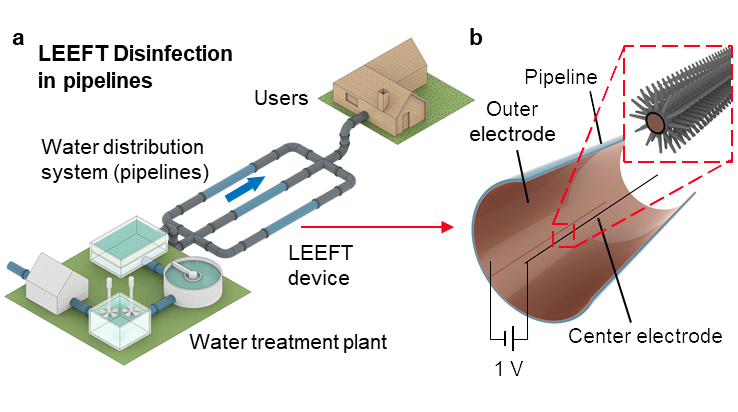 |
Photo 1. Electroporation on the cell membrane under a high external electric field.
|
Photo 2. Schematics showing (a) the LEEFT disinfection in pipelines and (b) the configuration of the coaxial-electrode LEEFT device.
|
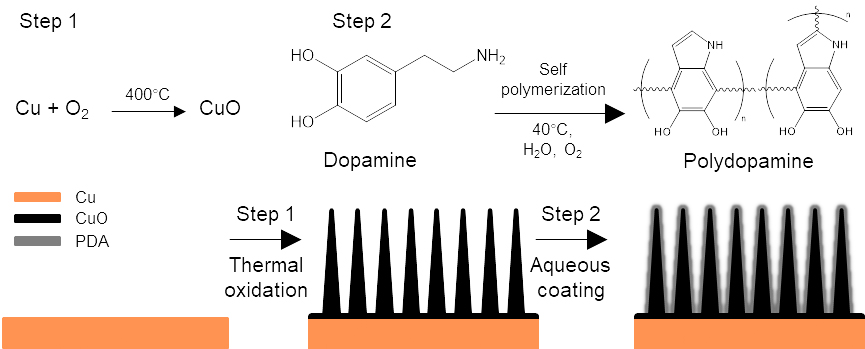 |
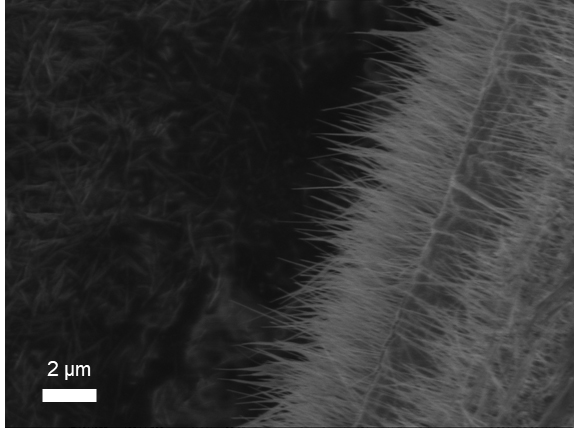 |
Photo 3. Schematics showing the fabrication processes of polydopamine-coated copper-oxide-nanowire-modified copper electrode. The polydopamine coating protect the nanowires and extend the life time of the electrodes.
|
Photo 4. Scanning electron microscopy image showing the bare copper-oxide nanowires on the electrode surface.
|
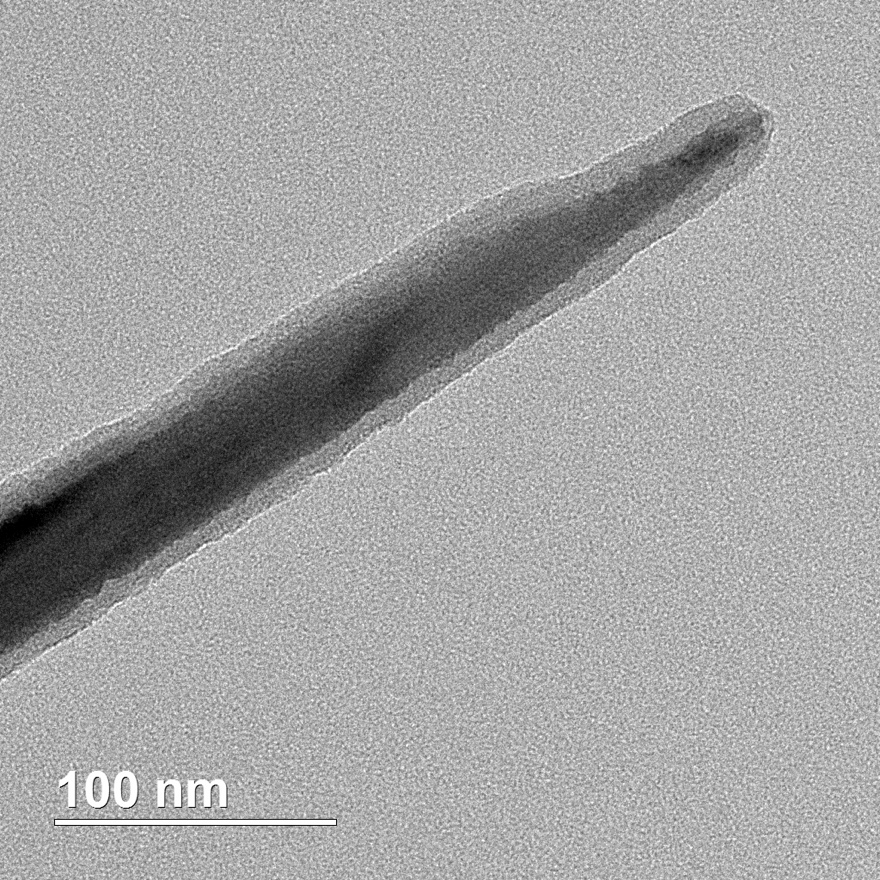 |
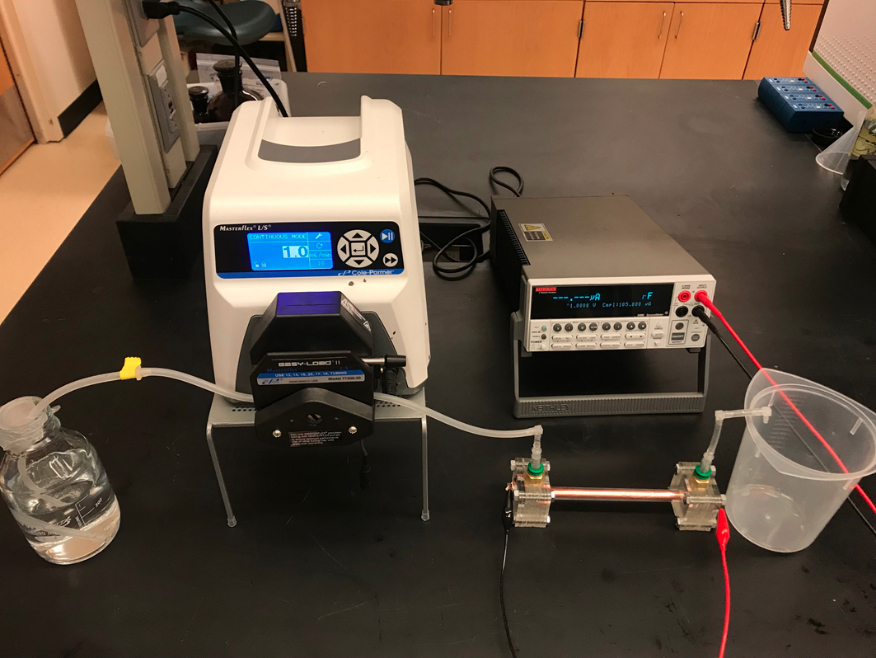 |
Photo 5. Transmission electron microscopy image showing the single copper-oxide nanowire coated with polydopamine.
|
Photo 6. Experimental setup of the LEEFT water disinfection.
|
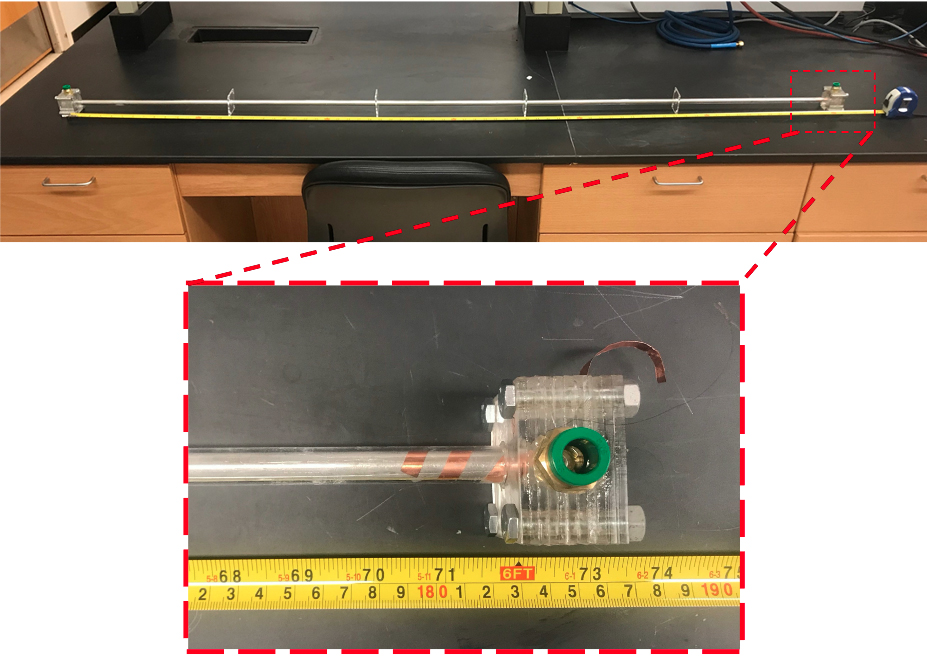 |
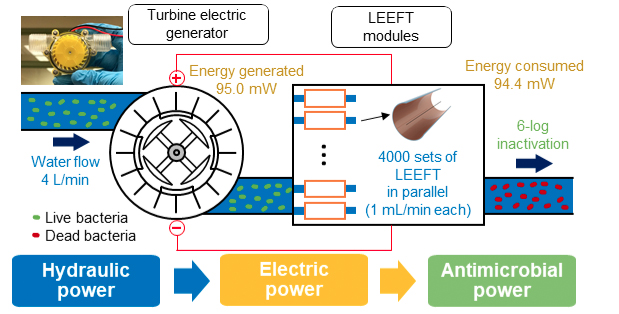 |
Photo 7. Photos of a 6-feet LEEFT device.
|
Photo 8. Powering the LEEFT devices with a turbine electric generator. When the water (4 L/min) flow through the turbine electric generator, the energy generated (95.0 mW) is sufficient to power the LEEFT modules (4000 sets with a flow rate fo 1 mL/min each) to disinfect the water.
|
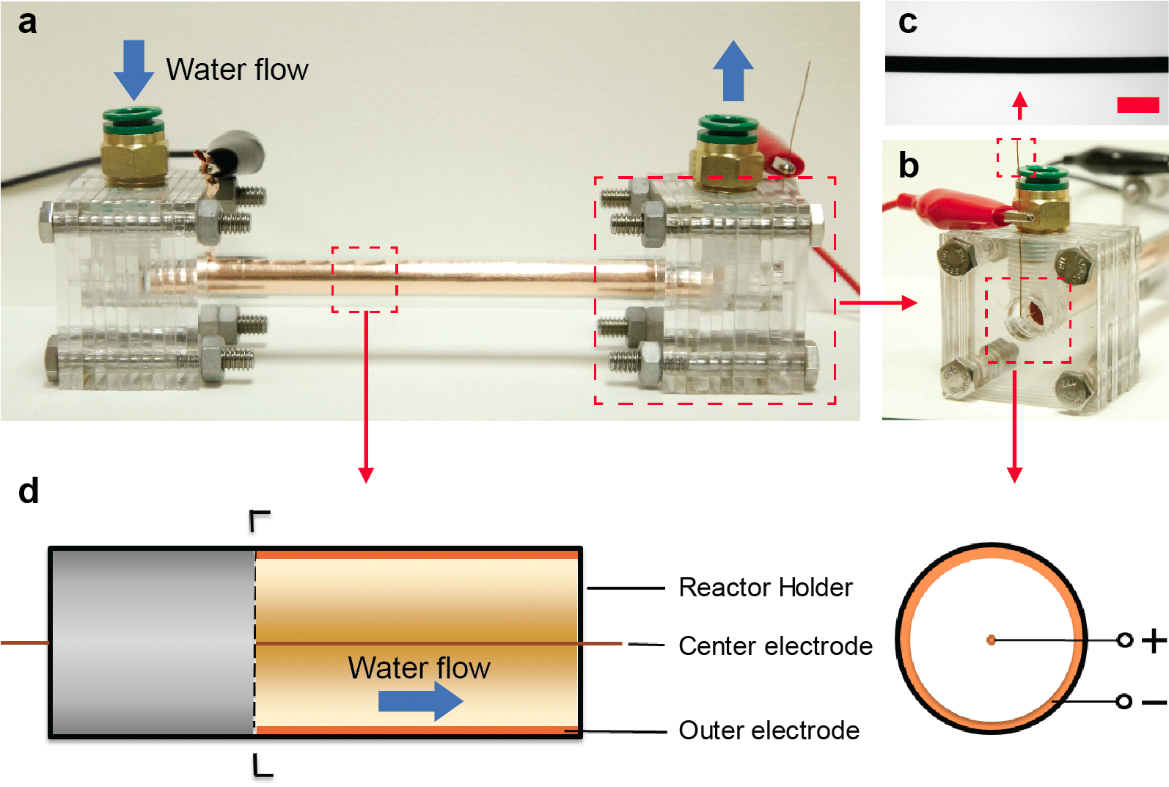 |
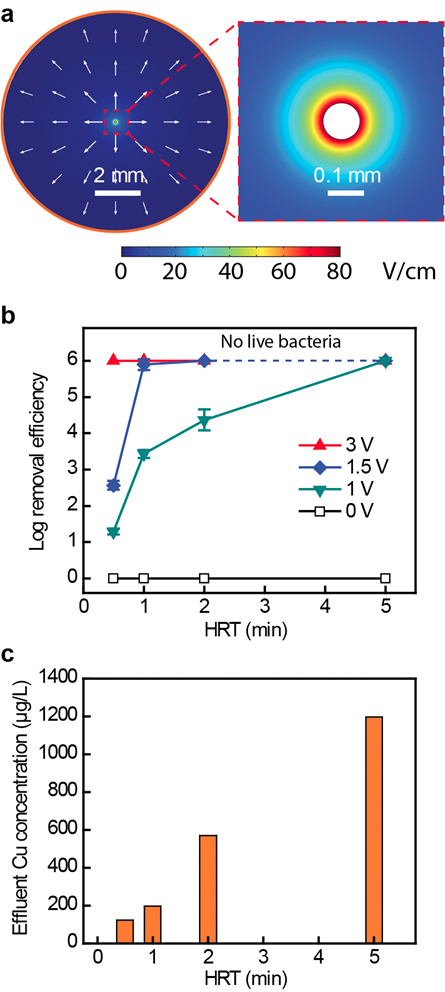 |
Photo 9. Coaxial electrode LEEFT-copper disinfection device. (a) Front view image of the device. (b) Side view image of the device, the center electrode used here is for exhibition only. (c) Optical microscope image of the center copper electrode, scale bar = 200 μm. (d) Schematic diagram showing the front view and cross-section view of the tubular reactor. The center electrode in (a) and (b) is for exhibition only because the electrode used in experiments (diameter = 76 μm) is too thin and soft to be visualized.
|
Photo 10. Non-uniform electric field and disinfection performance of the LEEFT-copper disinfection. (a) Electric field profile on the cross-section of the LEEFT-copper device simulated by COMSOL Multiphysics showing the non-uniform distribution of the electric field. The device is immersed by DI water and the applied voltage is 1.5 V. (b) Log removal efficiency of E. coli by the LEEFT-copper device operating at different HRTs and applied voltages. (c) Effluent copper concentration when the device operates at 1.5 V with different HRTs.
|
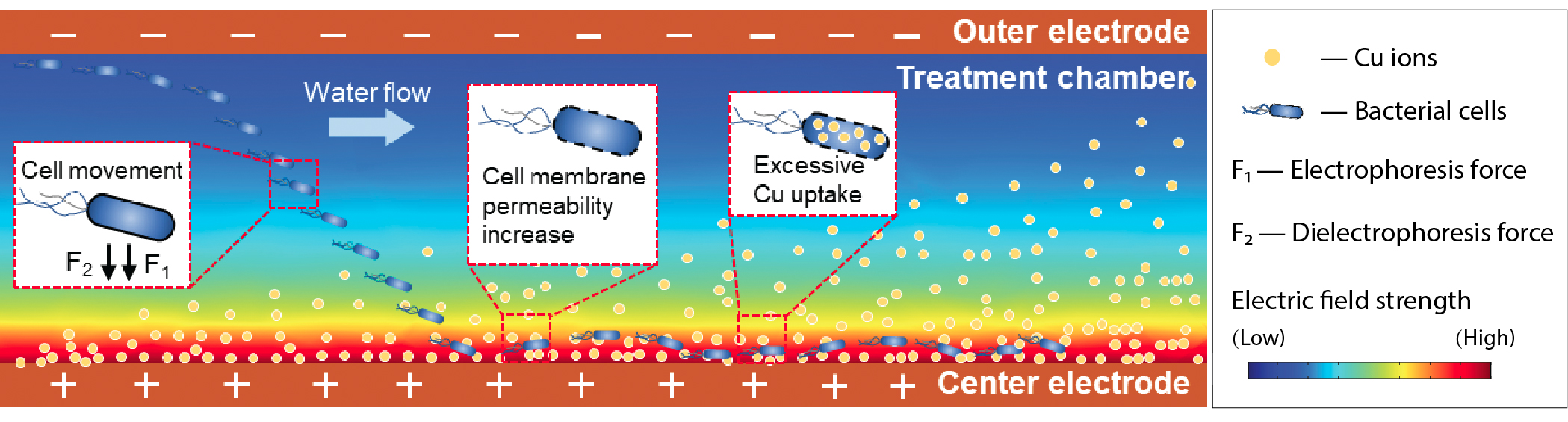 |
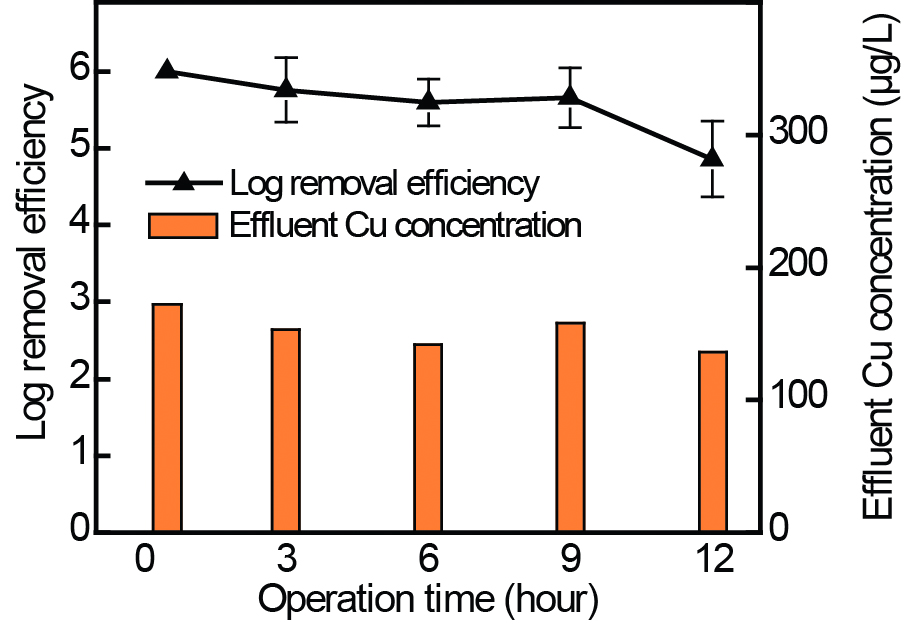 |
Photo 11. Schematic summary of disinfection mechanisms happened in the LEEFTcopper device.
|
Photo 12. Long-term disinfection performance of the LEEFT-copper device inactivating E. coli at 1.5 V and 1 min HRT.
|
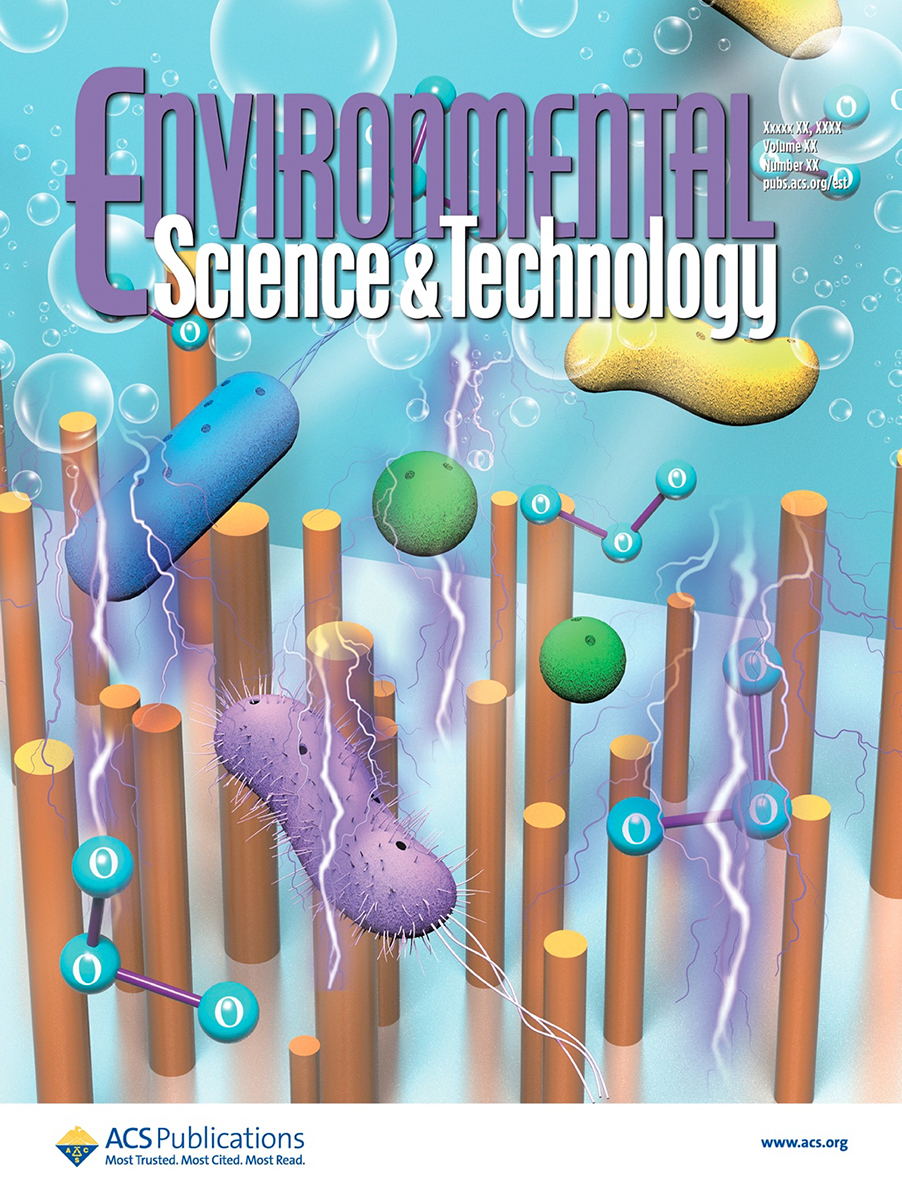 |
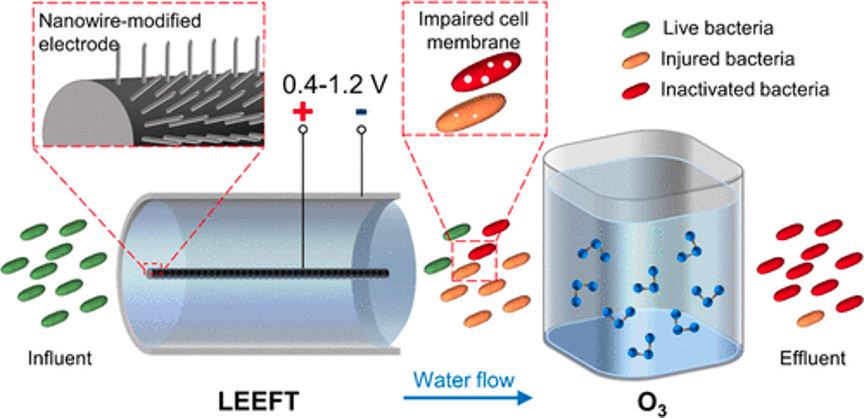 |
Photo 13. Supplementary cover of Environmental Science & Technology (November 3, 2020, Volume 54, Issue 21) highlighting Dr. Xie’s work on the LEEFT-ozone process (Locally enhanced electric field treatment (LEEFT) promotes the performance of ozonation for bacterial inactivation by disrupting cell membrane. Environmental Science & Technology, 2020, 54(21): 14017-14025).
|
Photo 14. Graphic abstract of Dr. Xie’s work on the LEEFT-ozone process (Locally enhanced electric field treatment (LEEFT) promotes the performance of ozonation for bacterial inactivation by disrupting cell membrane. Environmental Science & Technology, 2020, 54(21): 14017-14025).
|
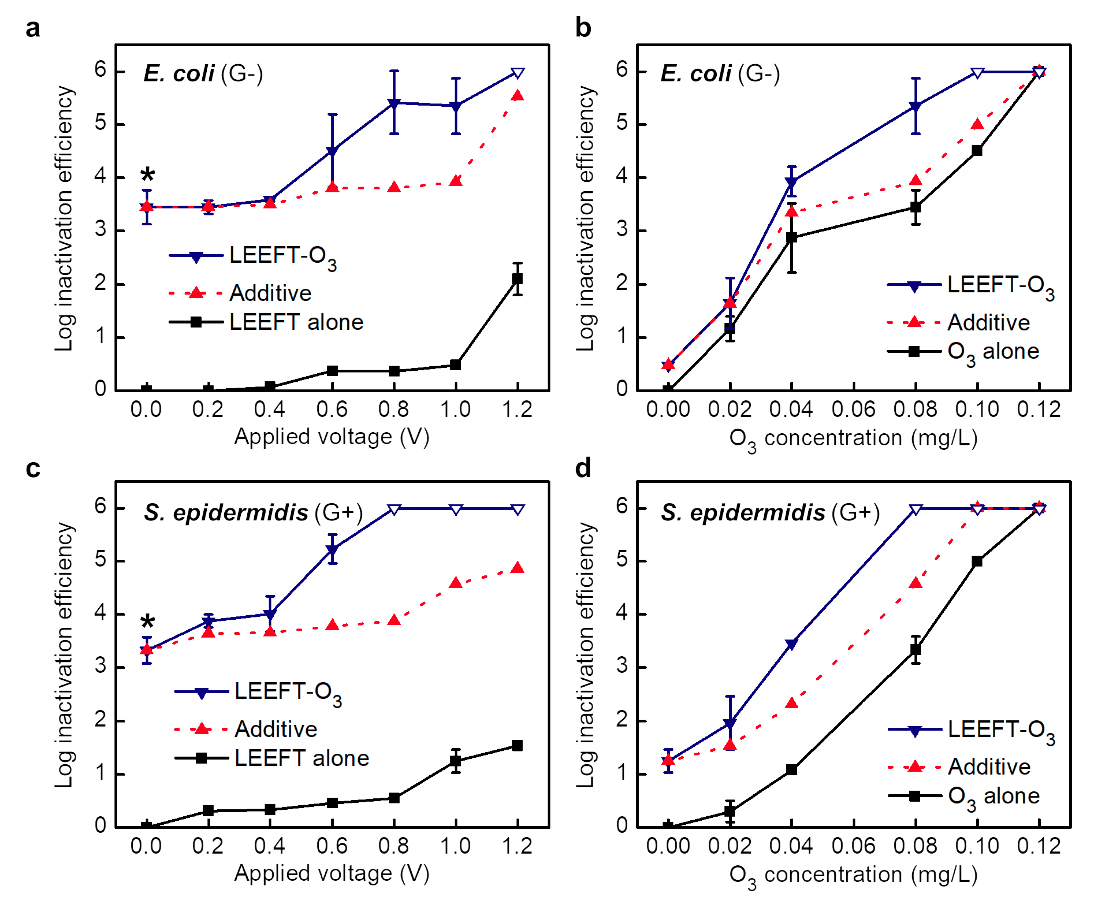 |
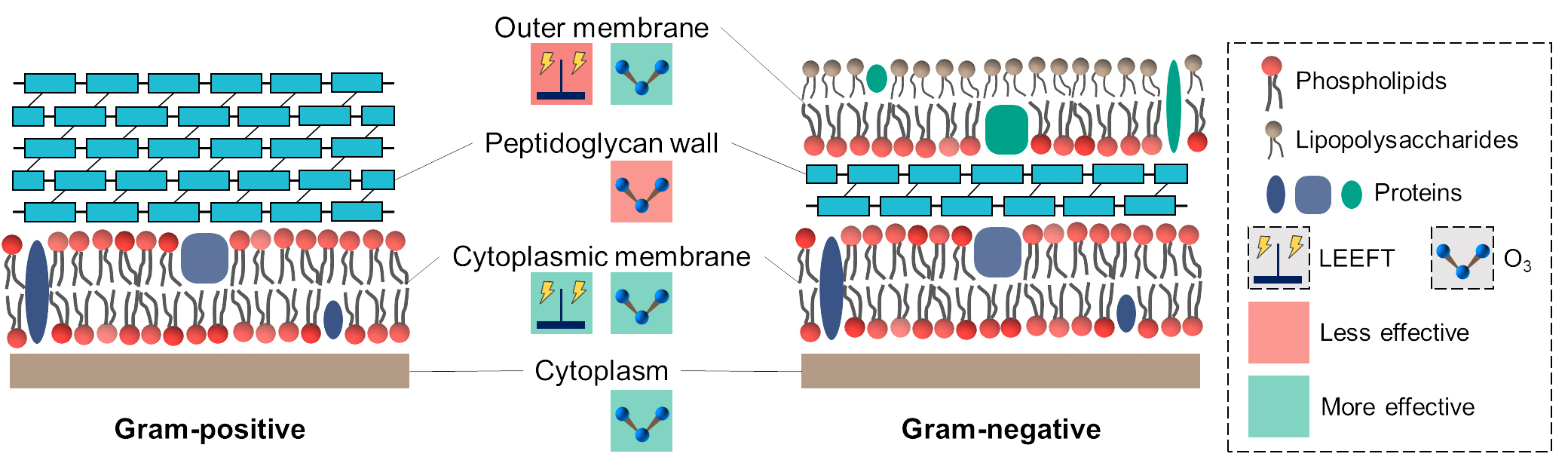 |
Photo 15. Enhanced disinfection effect using LEEFT-O3 process. (a & b) Log inactivation efficiency of E. coli with constant O3 concentration (0.08 mg∙L-1) and various applied voltage of LEEFT (a), and with constant applied voltage (1.0 V) in LEEFT and various initial O3 concentration (b). (c & d) Log inactivation efficiency of S. epidermidis with constant initial O3 concentration (0.08 mg∙L-1) and various applied voltage of LEEFT (c), and with constant applied voltage (1.0 V) in LEEFT and various O3 concentration (d). For the log inactivation efficiency, a solid symbol (e.g., ▾) indicates the exact value, while a hollow symbol (e.g., ▿) indicates an inactivation efficiency of >6-log (the detection limit), and no bacteria colony grew on the agar plates. The symbol “*” indicates the control experiments of the treatment by O3 only (0.08 mg∙L-1 dosage).
|
Photo 16. Illustration summarizing the disinfection mechanisms of LEEFT and ozonation against G+ and G- bacteria. The envelope of G+ bacteria consists of a very thick layer of peptidoglycan wall and an inner cytoplasmic membrane made of phospholipids. In Gbacteria, the peptidoglycan wall is much thinner, but is covered by an outer membrane, which is made of lipopolysaccharides on the external leaflet and phospholipids on the internal leaflet.
|
|